![Improvement Fastnesses and Color Strength Of Pigment Printed]()
INTRODUCTION:
Pigment printing is not only the oldest but also the easiest printing method as far as simplicity of application is concerned. The vast majority of printed fabrics have been printed with pigment dyestuff. Pigment printing has advantages such as ease of near final print at the printing stage itself, quality of the prints, applicability to almost every kind of fiber or mixture, and the ability to avoid any washing processes after fixation. [1, 2]
Soluble dyes, which have no affinity for any fiber, are used in a finely dispersed form. Film-forming binders are used to fix these pigments to the substrate by adhesion. The binders used in pigment printing are usually based on styrene-butadiene, styrene-acrylate or vinyl acetate-acrylate copolymers. [3] In the printing process, three dimension binder films have occurred in hot air ambient due to pH changing. Kind and amount of the chemical polar groups of fabrics influence fixation conditions and adhesion strength of the binder-to-fiber bond (important for rubbing fastness). Therefore, the effectiveness of coating binder affects the final properties of pigment printed fabric. [4]
Plasma treatments are ecologic, and can replace some finishing steps of textile materials. Plasma is produced by accelerating a gas via an electric field.Gas atoms are ionized, and free electrons are produced. Properties obtained with plasma are dependent on plasma parameters such as gas, discharge power, pressure, and electrical characteristics of the gas.[5,6] Plasma is the physicochemical treatment, and generally is used for changing surface properties of polymers or the other materials without any marked change in the bulk structure of the materials, because plasma only modifies outermost layers.[7-10] During a plasma treatment, several concurrent processes may occur at the plasma-polymer interface, depending on the chemical and physical characteristics of the plasma itself. As is well known, plasma treatment has two effects on the fiber surface. One is physical etching, which occurs when an inert gas, such as argon, is used to modify the surface. The other is chemical graft, which occurs when some polar radicals, such as oxygen and nitrogen, are induced to functionalize the surfaces of fibers. Crosslinking of radical and excited surface species may also contribute to the modification of the polymer surface structure. [11] Among the surface properties textile materials which can be improved with the use of the plasma are wetability and quality of dyeing and printing [5-12].
To achieve sufficient dyestuff adhesion and thus a satisfactory printing result, atmospheric plasma treatment has been applied to textile materials. In the past only a few articles, research reports, or some patents have been published referring to plasma treatment in textile printing application. [13, 14, 15] The present paper focuses on the printability of polyester fabrics and surface treatment as a way of increasing the surface energy (especially the polar component) to obtain a better printing result. Any surface treatment that involves the incorporation of polar groups, such as flame, corona or plasma treatment, may lead to deterioration in the water vapor barrier. Here, plasma treatment at atmospheric pressure was used, and surface energy of the substrate and printability were evaluated. After plasma treatment, improvement of the final color and fastness properties of the pigment printed fabric were examined. Correlations between the printability and the morphology and chemical structure of plasmatreated synthetic fabrics were also investigated. We were led to this application of plasma treatment during a study of the surface modification of polyester fabrics with plasma, when it became clear that functionalization of polymeric materials and micro-roughness by the plasma treatment rendered washfast printed fabrics. [16, 17] Morphological features of the surfaces were studied using AFM and SEM; the chemical composition was studied ESCA(XPS).
EXPERIMENTAL
Materials:
The specifications of the polyester (PES) fabric (plain weave) used this work are: warp 109 threads inch-1,80 den, weft 86 threads inch-1, 88 den. To minimize contamination, samples were extracted with acetone for four hours and dried at room temperature before being treated with plasma. C. I. Pigment Yellow 83(Figure 1) was kindly supplied by Dystar,Textilfarben, Germany and used for pigment printing.The binder Orgal ES 61, styrene-acrylic copolymer,and the synthetic thickener Orgaclear P 460, which is polyacrylic acid sodium salt, were kindly supplied by Organik Kimya, Turkey and used for the conventional pigment printing by thermal fixation.
Atmospheric Plasma Treatments (DBD-APT):
Dielectric barrier atmospheric plasma apparatus was investigated by Kocum and Ayhan. [18] An atmospheric plasma reactor operating at 50 Hz was employed. Argon (purity of >99.998) and air (20.9 %oxygen, 79.1 % nitrogen, and relative humidity < 3ppm) were used as plasma gases. The atmospheric plasma treatments were conducted in three different powers (50, 100 and 130 W) and treatment times (20,40 and 60 s). Because longer treatment time was not suitable for continuous textile dyeing and finishing process, maximum treatment time was determined as 60 s in pre-treatments. The distance between the electrodes was 2 mm, and the plasma reactor had 4 electrode couples. The samples were placed in the central position between electrode couple. Gas flowrate was adjusted to 103cm3/min.
Preparation of the Printing Pastes, Printing and Fixation Process The pigment paste was prepared from de-mineralized water, 5.8 % thickener, 15.5 % binder and 3.25 % pigment dye. For printing treatment, a 70 mesh flat printing screen and 10 mm diameter squeegee were used. Printing speed was 4 m/min and pressure grade was 1. A Johannes Zimmer Type MDK Laboratory machine was used for printing. For drying and curing,a Mathis Laboratory dryer machine was used and the printed samples were dried and cured at 100 ?C for 10 minutes.
Printing was applied to rectangular fabrics (10 x 30 cm). The printed fabrics were evaluated according to standard test methods to determine if atmospheric plasma treated fabrics had equivalent or improved performance properties compared to untreated fabrics.
MEASUREMENTS
Surface Energies
The surface energy was determined by measuring the angle of contact using the sessile drop method (KSV CAM 200, Izmir, Turkey). The liquids used for calculating surface energy according to Fowkes were water, ethylene glycol, diiodomethane, and formamide of known γp (polar coordinate) and γd (disperse coordinate). [19]
The surface energy of the solid has two components, namely, a polar component (γsp) and a disperse component (γsd). Both components contribute to the total surface energy. The polar component is important to understand the hydrophilic surface modification, whereas the disperse component indicates the changes in hydrophobic modification or formation of a cross-linked network. The measurements of this study were performed at room temperature and 65 % relative humidity one day later after plasma treatment.
Color
The reflectance measurements of the printed samples were measured by a Minolta 3600d spectral photometer (Konica Minolta, Japan) under the following condition: D65/10? measurement geometry,measurement wavelength range from 400 nm to 700nm, measurement area of 113 mm2, and SIN-specular included measurement mode. Color strength values(K/S) of the dyed samples were calculated by the Kubelka-Munk equation [11] by using Minolta 3600d software.
Fastness
The dye color fastness tests to washing, light and rubbing were evaluated by ISO 105-C06, ISO 105-B02 and ISO 105-X12, respectively. [20-22] SDC(Swiss Agency for Development and Cooperation) multi-fiber strip fabric as an adjacent material was used.
Analytic Procedure
An X-ray Photoelectron spectroscopy for chemical analysis (ESCA/XPS) was used to assess the chemical composition of the surfaces (METU,Ankara). The instrument employed was a SPCS XPS with a Mg Ka X-ray source with energy of 1253.6eV. The instrument was calibrated against gold (84.0eV). The take-off angle was 45°. In this study, the measurement depth was 7 nm, and analysis chamber pressure was 10-8 to 10-9 torr. Surface Morphology The microstructure of PES woven fabric before and after plasma was characterized by using SEM (Scanning Electron Microscopy) a Philips XL30S FEG Microscope (Izmir Institute of High Technology, Izmir) with digital microscopy imaging.
Surface morphologies of the treated and untreated samples were studied by using a MMSPM Nanoscope IV Atomic Force Microscope, AFM,(Izmir Institute of High Technology, Izmir). It has a probe that has a nanosize tip mounted on a flexible cantilever. The tip is scanned slowly across the surface of a specimen. The force between the atoms on the surface of the scanned material and those on the scanning tip cause the tip to deflect. This deflection can be recorded by using a laser focused on the top of the cantilever and reflected onto photo detectors. The photo detector signals are used to map the surface characteristics of specimens with resolutions down to the nano-scales. Atomic force microscopy provides high resolution images of surfaces even if they are non-conducting. In this study, scanning was carried out in tapping mode. All images were obtained at ambient conditions one day later after plasma treatment.
RESULTS
The Effects of Treatment Time and Power on Surface Energy, K/S Values and Fastness of
Pigment Printing
To study the influence of treatment time and power, plasma treatments were carried out at 50, 100 and 130 W working powers for 20, 40 and 60 sec durations times. The pigment printing was carried out one day after plasma treatments and results were summarized in Table I.
Surface energy and K/S values were increased with increasing of plasma exposure time and power.Explanations about increasing surface energy were given in Reference 23. This can be attributed to the increasing number of plasma created polar groups (an increase of the surface energy means increasing polar groups) and roughness on the surface, due to etching and other chemical changes of the surface. As can be seen in Table I, the best color strength was obtained when the PES fabric was treated 130 W-60 s argon or air plasma. Overall, the printability after plasma treatment was quite acceptable. And increasing printability was reported some researchers. [23, 24] The rubbing fastness depends on binder elasticity, adhesion on the textile materials, and uneven dispersing of the pigment in the print paste. [25] Assuming that binder was well dispersed and had the same elasticity, these results indicated that plasma treatment improved the fabrics adhesion to printing paste and also binder. These rubbing results (Table I) can be attributed to better binder film strength of plasma treated fabrics than untreated fabrics. After plasma treatment, amount of linkage between binder and fibers and resistance of the chemical bonds increased because of increasing polar groups, so, especially rubbing fastnesses of treated samples increased. All K/S values increased after plasma treatments of over 20–60 s durations. The best treatment condition was 60 seconds at 130 watts for both argon and air plasmas.
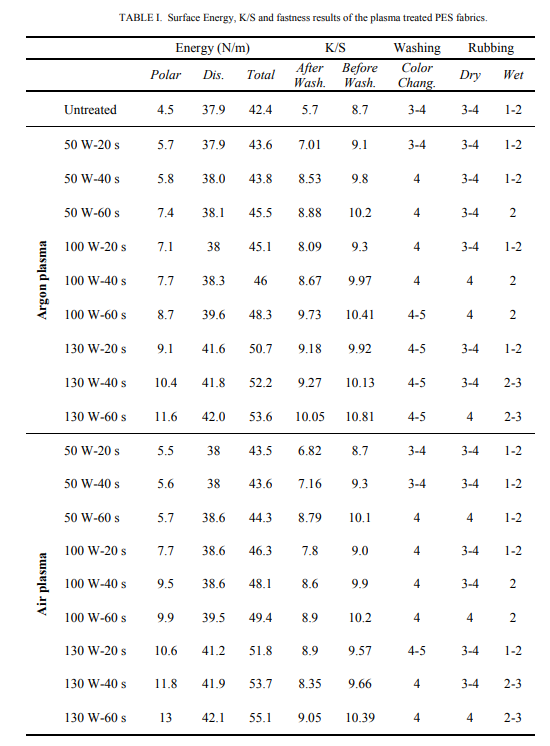
Analytic Procedure
XPS results of untreated and modified PES by argon and air plasma treatment in the optimum condition were given in Table II and Figure 1.
From Figure 1 and Table II, it is concluded that a decrease in the C (1s) peak was observed (from 73.3 to 63.3%) when PES fabric was treated in air plasma for 60 s. At the same time, the O (1s) peak increased (from 26.6 to 35.2 %). When PES fabric was treated in argon plasma for 60 s, a decrease in the C (1s) peak was observed (from 73.3 to 64.8 %), but the O (1s) peak increased (from 26.6 to 64.1 %). The O/C ratio of the untreated, argon and air plasma treated PES increases as follows, respectively: 0.363<0.526<0.556. Increasing O/C atomic ratio means that oxygen-containing functional group amount increases. Increasing of oxygen and nitrogen amount has resulted from environmental air (in laboratory) after plasma.
When treated with air plasma, the concentration of oxygen and nitrogen found on the surface is greater than when the fabric is treated with argon plasma.
This difference explains the improvement in printability after air plasma treatment.
Analysis of Surface Morphology SEM image was observed to comprehend the alteration of surface morphology of treated fabrics.
The PES fiber surfaces after argon and air plasma treatment are shown in Figure 2 with a magnification of 10.000. It can be seen that the surface-etching process occurs on the surface of argon and air plasma treated fiber.
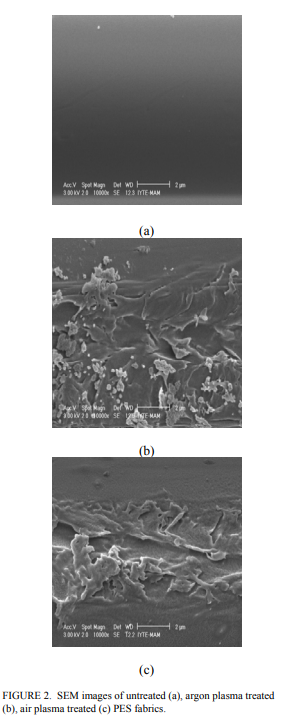
Figure 2(a) shows the SEM image of untreated PES fiber, while Figures 2(b) and 2(c) display the SEM images of plasma treated PES fiber with argon or air gases, respectively. Figure 2(a) clearly demonstrates that the untreated fiber surface is smooth and free from roughness, indicating that no damage occurs on the fibers surface. However, Figures 2(b) and 2(c) illustrate a change in the fiber surface morphology. When compared with the argon plasma treatment, the air plasma treatment has a more severe damage on the fiber surface. This is probably due to the comparatively slow rate of physical etching introduced by the argon plasma gas. [26] In summary, The topography shown in Figure 3(a) was typical for untreated PES specimens. A visual qualitative analysis clearly highlights changes in the textile Surface topography after the plasma treatments. The surface of the untreated PES fabric was smoother than plasma-treated samples. The effect of the plasma treatment caused an increase in surface roughness of the sample. The AFM images of treated samples showed a progressive change of the PES surface topography with the formation of an extensive array of bumpy features. On the relatively smooth surface of the untreated PES textile, the plasma treatment creates pits and micro-pores whose density, depth and size change as a function of plasma condition.
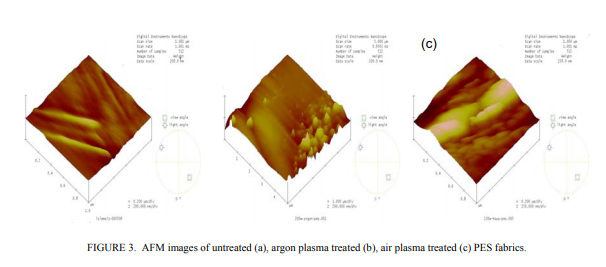
Changes in the surface roughness of the plasma treated PES fibers were quantified by root-mean square (rms) values to highlight the surface modifications due to argon and air plasma treatments.Rms roughness data, given as standard deviation over all height values within the surface area of interest,were determined from 1 μm2。
AFM images. The rms
values were given in the Table III. For the PES fibers, the roughness increased from 9.2 nm for the untreated sample, reaching a value of 16.1 and 20.8 plasma treatment with air gas is more efficient because air has oxygen molecules. It also signifies that a stronger porous surface effect is created on the air plasma-treated PES fibers.
The AFM analyses performed on plasma-treated samples, which the highest K/S values were obtained, and the AFM images of the untreated and plasmatreated PES fabrics have been shown in Figure 3. All images are 10,000 °A ×10,000 °A, with the corresponding z ranges given above the individual images. nm after argon and air plasma treatment, respectively. Progressive pitting and surface damage of the treated samples suggested that the surface area also was increased significantly.
While using air plasma treatment, the etching effect made the surface of fibers even rougher because of containing oxygen.
Plasma discharge interacts in numerous ways with a material surface. At rather severe conditions, the polymer surface is subject to etching effects, whereby the polymer is continuously degraded. While chemical etching is promoted by radicals as in O2, CF4 and SF6 discharges, physical etching occurs under strong ion bombardment, as for example in argon plasma.
CONCLUSIONS
The printability of the textile materials depends on the surface characteristics and functional surface properties of fibers. An important result of this study was that the properties could be further enhanced by a pre-treatment of the substrate itself, such as plasma treatment before the pigment printing stage. Surface area and surface polarity increased with plasma treatment. The surface treatment raised the surface energy (especially the non-dispersive component) and this gave a more favourable interaction with binder. In addition, etching was assumed to remove weak boundary layers (e.g., impurities) from the surface. Because of etching, roughness of polymeric materials increased after the air and argon plasma treatment, so surface area that was bonding binder and also dye molecules increased. The aim of this work was to optimize the treatment process, and to study its effects on printability. PES fabrics printed with pigment dyestuff after argon and air plasma treatment had higher color intensity than those untreated fabrics printed by the same method at equal pigment color ratios. Fastness of treated samples was also higher than untreated fabrics because of improving bonding strength to the binder. At the same time, more color and dry rubbing fastness can be obtained with high treatment power argon plasma treatment. Therefore, we recommend proper argon or air plasma treatment to improve the functionality of the textile fibers and also fabrics.